Flow-induced vibrations in piping systems are a critical aspect of mechanical engineering, impacting the reliability and integrity of various industrial setups. The Japan Society of Mechanical Engineers (JSME) provides a helpful classification for these vibrations, enabling engineers to understand the different types and interactions with piping systems.
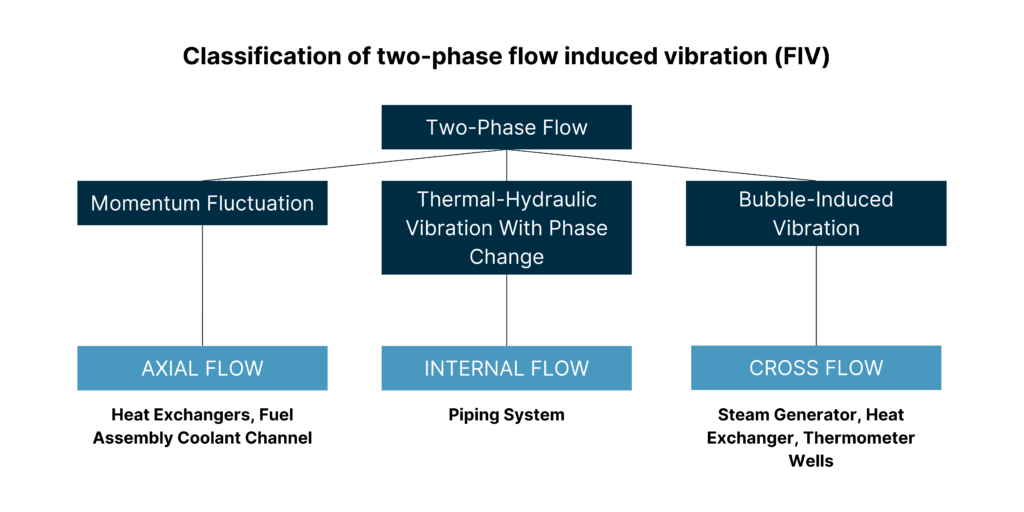
Excitation Mechanisms
JSME’s classification identifies three primary excitation mechanisms:
- Momentum Fluctuation: Changes in density within piping systems result in impact loads, causing fluctuations in piping vibrations. This mechanism is particularly significant in scenarios involving density changes due to fluid flow.
- Thermal-Hydraulic Vibration with Phase Change: This category encompasses energy transport between gas and liquid phases, including phenomena like boiling, condensation, and chemical processes. These processes can induce vibrations within piping systems, especially affecting internal components.
- Bubble-Induced Vibrations: The presence of unstable bubbles within fluid flow can lead to interactions with piping, resulting in vibrations. Variations in bubble sizes and flow dynamics contribute to this type of vibration excitation.
Types Of Interactions
JSME’s classification also describes three types of interaction with piping systems:
- Axial Flow: This occurs when a two-phase flow surrounds the piping in the axial direction. Examples include heat exchangers where bubbly flow induces vibrations in the piping.
- Cross Flow: Similar to axial flow, but the flow direction is in the lateral direction of the tubes in heat exchangers. Cross flow induces vibrations in the piping due to interaction with the fluid.
- Internal Flow: Refers to two-phase flow inside the pipe, interacting with the pipe and causing vibrations. This type of interaction is crucial for ensuring the reliability of power or process piping systems.
Characteristics of Two-Phase Flow Regimes
Understanding the characteristics of two-phase flow regimes is vital for predicting and mitigating flow-induced vibrations. Various flow regimes, such as stratified smooth flow, elongated bubble flow, and slug flow, exhibit distinct behaviors that influence piping vibrations.
An important aspect of flow-induced vibrations lies in slug flow phenomena, characterized by short yet impactful slug formations within piping systems. These slugs exert dynamic forces on individual piping components, potentially leading to resonant vibrations and structural stresses. Calculating these forces requires the consideration of parameters like slug velocity and length, crucial for assessing system response and mitigating vibrational risks.
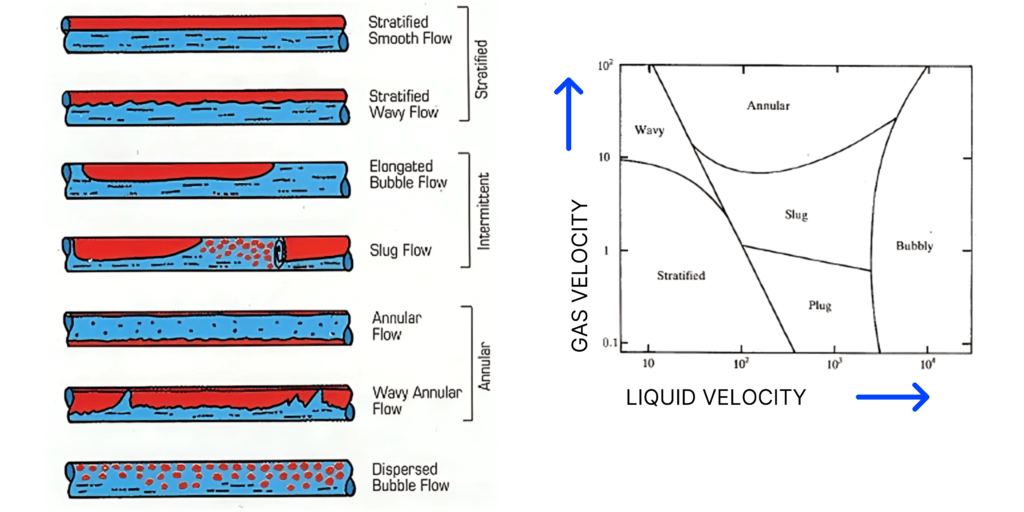
Engineering Approaches
Dealing with flow-induced vibrations during both the design and operation stages requires careful consideration and proactive measures. Engineering approaches include:
- Eliminating Low-Frequency Eigenmodes: Adding supports to absorb slug forces and prevent resonance-induced vibrations.
- Avoiding Slugging Regime: Designing systems to minimize two-phase flow occurrences.
- Detailed Mechanical Response Analysis: Conducting thorough assessments based on vibration measurements to evaluate stress levels and fatigue risks.
During operational stages, robust monitoring and assessment protocols are essential for detecting and managing flow-induced vibrations. Utilizing vibration-based limits and conducting detailed mechanical response analyses based on measurements enable proactive management of vibrational risks. In this scenario, practical challenges, such as limited information availability and thermal expansion considerations, highlight the complexity of addressing flow-induced vibrations in operational contexts.
In addressing flow-induced vibrations, engineers must navigate the balance between structural flexibility and rigidity, optimizing designs for both static and dynamic conditions. By integrating comprehensive understanding, proactive design measures, and robust operational protocols, industries can effectively manage the complex challenges posed by flow-induced vibrations in piping systems.
Case Study
The case study below, involving a hydrogen cyanide ammonia stripper, illustrates the practical application of engineering solutions to address flow-induced vibration issues. Engineers can effectively mitigate the risk of fatigue failure in piping systems through vibration measurements, detailed mechanical response analysis, and implementation of corrective measures.
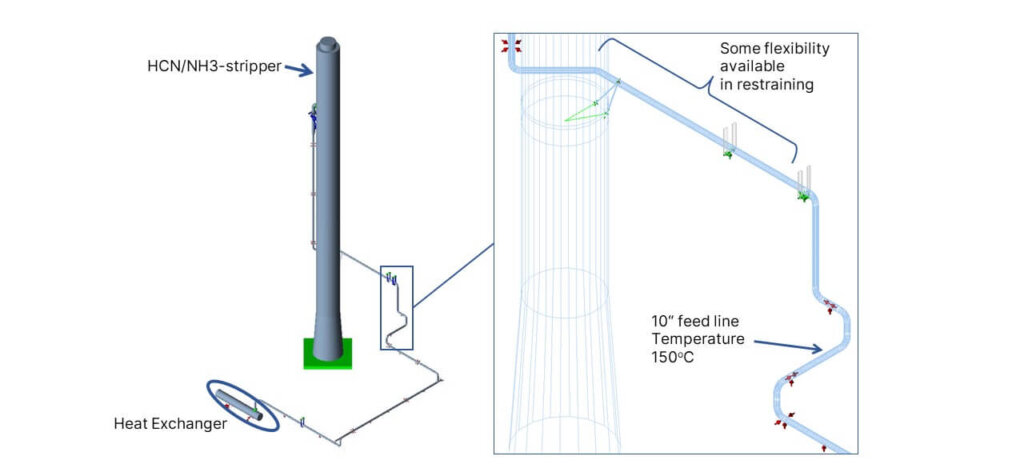
The Feed Line System
The feed line, originating at the heat exchanger and terminating at the stripper, underwent detailed assessment. This examination involved a comprehensive analysis of the system’s structural components, including steel structures and support attachments. Unlike static assessments, dynamic analyses required realistic friction coefficients and meticulous modeling of eigenmodes to accurately predict system responses.
Identification Of Failure
A fatigue failure was detected above an elbow in the feed line. Vibration measurements revealed frequencies between 4 and 9 hertz, prompting further investigation into potential causes and implications.
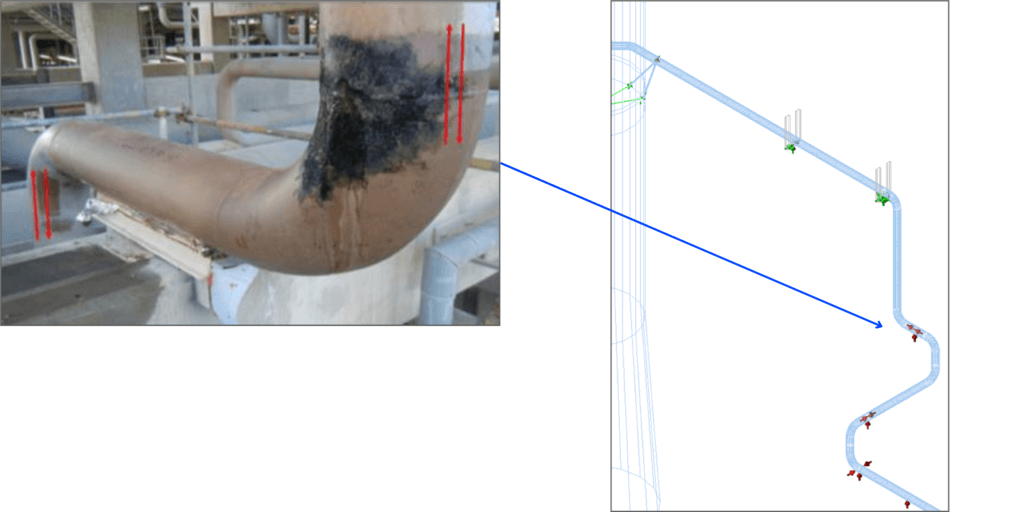
Mechanical Response Analysis
The team conducted a detailed mechanical response analysis, considering slug forces provided by the client and their impact on the system. Through time history analysis, resonance modes were identified near the failure location, aligning closely with field observations.
Stress Assessment
Stress amplitudes were calculated using industry-standard methodologies and compared against failure curves. Results indicated stress levels approaching or exceeding endurance limits, emphasizing the criticality of addressing the issue promptly.
Mitigation Strategies
To mitigate low-frequency eigenmodes and prevent future failures, the team recommended restraints at strategic locations. Implementation considerations included static stress assessments, load evaluations, and operational constraints. In one instance, a clamp with packing was applied to the leaking elbow without interrupting system operation.
Challenges And Considerations
Implementing support measures during system operation presents unique challenges. Factors such as geometry, displacement severity, and support rigidity influence the selection and installation of solutions. Minimizing clearances between pipes and supports is essential to prevent hammering effects and ensure effective load absorption.
Lastly, this case study underscores the importance of proactive maintenance and rigorous analysis in preventing fatigue failures in industrial systems. By understanding system dynamics, conducting thorough assessments, and implementing appropriate mitigation measures, engineers can safeguard operational reliability and worker safety in complex industrial environments.
Conclusion
Engineers face various challenges when analyzing flow-induced vibrations, including obtaining accurate excitation mechanism data, matching models with field measurements, and balancing static and dynamic solutions. Practical issues such as support implementation during operation and minimizing gaps between piping and supports are crucial for ensuring effective vibration control.
Flow-induced vibrations pose significant challenges in maintaining the reliability and safety of piping systems. By understanding the excitation mechanisms, types of interaction, and engineering approaches, engineers can proactively address flow-induced vibration issues, ensuring the integrity and longevity of industrial piping systems.