Hydrogen generation equipment has an inherent risk for internal explosions, posing an issue to safety. The hydrogen piping and equipment are required to withstand internal explosions by limiting the damage to deformation. Although this disqualifies the component for future use, safety is not compromised.
The objective was to find a DLF to statically evaluate the internal hydrogen explosion. The plastic deformation caused by the explosion was determined using FEA. The equivalent static pressure that would yield the same plastic strain, provided the basis to determine at what values the pressure tests will need to be performed.
Introduction
Our client is a global company engaged in building end-to-end green hydrogen generation systems with a capacity between 1 and 10MW.
These units work through an electrolyzing process, confining in the same stack, oxygen and hydrogen at different compartments. Therefore, it is impossible to exclude the possibility of hydrogen entering the oxygen side in case of failure within the stack. This would cause an explosive mixture of hydrogen/oxygen in the chamber.
Due to the existence of ignition sources, a potential risk of explosion is considered inherent to the system. This danger of explosive mixtures produced in the system poses a challenge to ensure its safety. This requires proof that its components and equipment can withstand internal detonation shock waves.
With Dynaflow’s experience in complex problem solving and analysis for pressure equipment, they were the choice to help us solve our problem.
Customer Testimonial
Background
Given the extensive experience in complex dynamic assessment of piping and equipment, DRG was tasked with finding a correlation between the data obtained from the explosion tests and simulations performed by the client and static pressure values that help prove compliance through static pressure tests.
The initial focus of this project was to obtain an analytical understanding, through a literature study, of how sudden explosion peak pressures and subsequent impulses affected the equipment and components dynamically. The goal was to translate the explosive pressure impulses to a DLF (dynamic load factor) that would be used as a factor to convert the dynamic explosion pressure to a static pressure that yields similar mechanical strain as the moving wave front. This equivalent pressure will be used to prove that the complete system complies with the requirements needed for explosion safety.
Further to discussions with the client and the lack of accuracy in existing academic research to address this problem and obtain reliable DLF values, DRG shifted to numerical analysis of the internal explosion using FEA (Finite Element Analysis).
The complexity lies in the quantification of a DLF to convert the dynamic explosion pressure to a static pressure that yields similar mechanical deformation. This required a sophisticated approach, integrating advanced computational methods and experimental data.
Daniël van Baalen, Project Manager & Head of Renewables
Solution
To capture the entire explosion wave in the analysis, different piping components were assessed under different explosion signals using various FEA models. The plastic strain obtained from the dynamic simulation was used as a target value to find the required equivalent static pressure for the different geometries and impulses.
The FEA model was required to capture the mechanical behavior of different piping components (straight pipes, elbows, and tees) through internal explosions produced by the ignition of the hydrogen/oxygen mixture. The results from the FEA analysis provided a better insight into how the dynamic application of the explosion differs from the static loading of the system and how to correlate both for explosion-proofing of the equipment.
The dynamic model includes the shockwave as a moving pressure front along the component geometry, with a sudden pressure peak that linearly decreases to a sustained pressure value in the order of milliseconds.
Results show the importance of conducting accurate FEA of the event, as the sudden pressure increase excites some high-frequency eigenmodes that travel through the pipe wall. These vibrations produce reflections that can meet back with the moving wavefront, creating amplified local bending moments, observed as high plastic deformation rings. Although the exact vibration pattern depends on the exact system geometry, the resulting stress and deformation will be similar.

The FEA approach helped to capture the localized plastic strain areas that were subsequently used as target values to find the equivalent static pressure. Using an analytical approach combined with FEA the static internal pressures were determined to yield the same peak strain values of the different components. This method ensures testing of the equipment against the most critical stress conditions.
Conclusion
The goal of the FEA analyses and subsequent calculation of equivalent static pressures was to verify the integrity of piping components, gaskets, and more complex equipment of the system through static pressure tests.
The simulation results showed that the required equivalent pressures for components with geometrical discontinuities were reduced in comparison to a straight pipe. The Stress Intensification Factors (SIFs) applicable to those areas limit the required static pressure in that component to yield the same stress and strain values. This is the reason why it is recommended to test gasket sealings and equipment with the components that require the highest equivalent pressure to “mimic” the results of the explosion analysis, i.e. the straight pipe, neglecting the SIFs.
This analysis methodology provides reliable insight for further proofing of equipment. It also gives a clear understanding of the possibilities and potential concerns of equivalent static pressure tests for explosion events.
With the results from Dynaflow’s work we have that required step further in our analysis of the overall problem to get to final conclusions. When more analyses for this subject are needed in the future, we will certainly get into contact with Dynaflow again.
Customer Testimonial
At Dynaflow Research Group, we're dedicated to supporting renewable-related projects tackling complex engineering challenges. Our commitment involves innovation, collaboration and using the collective expertise of our team to advance sustainable solutions for a cleaner future.
Daniël van Baalen, Project Manager & Head of Renewables
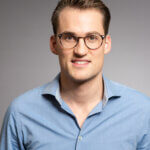